Gene Therapy for ALS
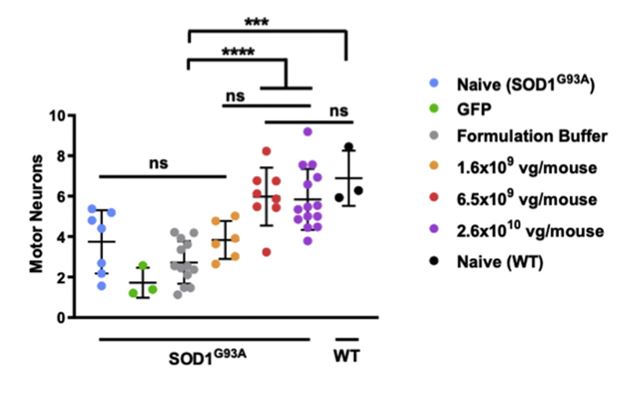
The Boulis lab has focused on using gene- and stem-cell-based therapies to treat ALS. Most ALS treatment centers on protecting motor neurons and keeping them connected to muscle cells. One way of achieving this is to use molecules known as neurotrophic factors. These proteins promote the survival of motor neurons and encourage neurons to maintain or create new connections with muscle cells. Recombinant factors, i.e., those made in the lab, have been efficacious in animal models of ALS. However, it has been difficult to deliver them at therapeutic levels in clinical trials. Allowing a patient to produce their own supply of neurotrophic factors is one possible solution to this problem. Gene therapy allows researchers to turn a patient’s own cells into trophic factor factories, bypassing the need for repeated delivery of recombinant factors. In collaboration with Dr. Nicholas Maragakis at Johns Hopkins University (https://cmm.jhmi.edu/index.php/cmm-faculty/nicholas-j-maragakis-md/), we evaluated a gene therapy vector that expresses the neurotrophic factor, neurturin. This therapy is injected directly into the spinal cord, where it generates local production of this factor. When injected into the cervical spinal cord of a mouse model of ALS, this therapy slowed the loss of motor function and prevented the loss of motor neurons in a dose-dependent fashion. Although not a cure, this approach appears to be a promising treatment to slow down the advancement of ALS, giving patients a longer period of quality life.
Intrathecal Gene Delivery
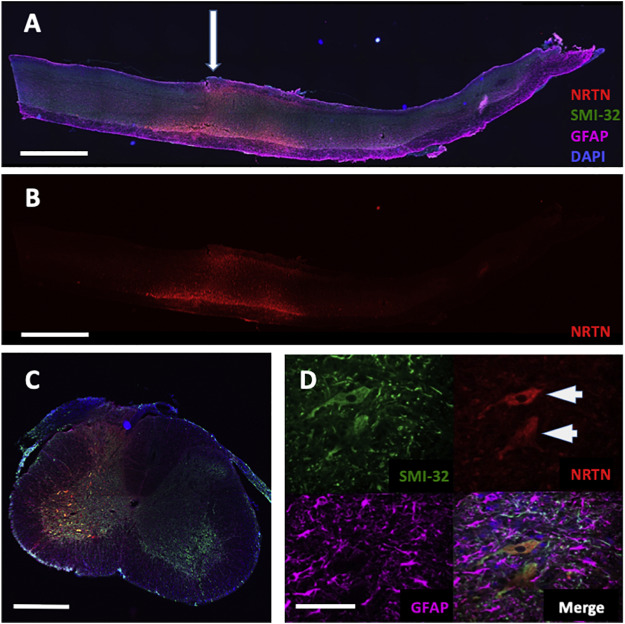
The approach used for the neurturin gene therapy above has some important limitations. Since it needs to be injected directly into the spinal cord, the procedure is very invasive. The bony lamina that protect the spinal cord must be removed, and the cord is injected multiple times at regular intervals. This approach can only be used to treat small regions of the spinal cord, though. Therefore, one must focus treatments on the most important motor functions: breathing and the arms and legs. This approach is not ideal because it allows the disease to advance unabated in the rest of the body.
One potential solution to this problem is intrathecal gene delivery. In this approach, the gene therapy vector is delivered into the fluid that surrounds the brain and spinal cord, the cerebrospinal fluid (CSF). Some, but not all, vectors are able to reach the motor neurons from the CSF. We previously discovered that one particular vector, known as AAV9, has this ability. When injected intrathecally, AAV9 is able to transduce motor neurons all along the entire length of the spinal cord. However, as the lab pursued this technology as a way to deliver neurotrophic factors to the spinal cord, we discovered that not all lots behave the same way. It is currently unclear what the difference is between lots, but we have hypothesized that it is related to small differences in the manufacturing process. We are currently seeking funding to investigate the underlying differences. This would allow us to design more potent vectors for the treatment of ALS and other neurodegenerative diseases.
Intrathecal Riluzole for the Treatment of ALS
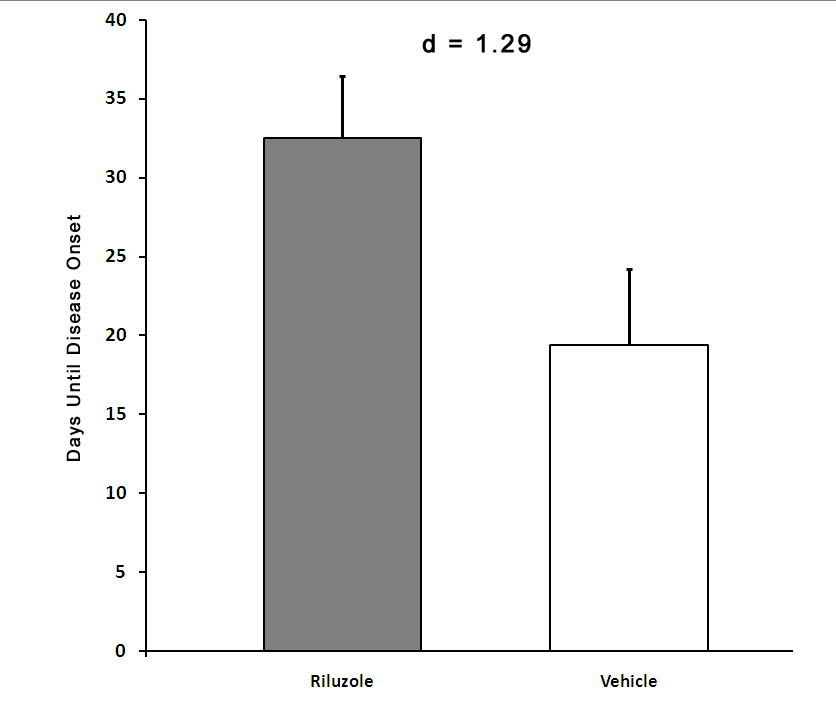
Riluzole is one of only two approved treatments for ALS, but its efficacy wanes as the disease progresses. Evidence from patients with ALS and animal models of the disease suggest that changes occurs in the blood vessels in the brain and spinal cord. These changes make it more difficult to get the drug from the blood stream to the motor neurons. We have hypothesized that delivering riluzole directly to the CSF will increase the drug efficacy and may lead to a more pronounced slowing of disease progression and/or to an increased survival.
We have developed an intrathecal delivery approach using an implantable pump. This system provides continuous delivery of riluzole to the CSF. In a large animal model, riluzole concentrations in the spinal cord achieved with this approach were as much as 10-times higher than levels achieved by oral administration of this drug, and there the significant side effects observed with higher oral doses in humans were not observed. A small number of patients have already received this treatment as an off-label therapy. We are currently trying to secure funding for a Phase I clinical trial for this approach and develop new tools to optimize drug delivery.
MRI-guided, Minimally Invasive Delivery of Therapeutics to the Spinal Cord
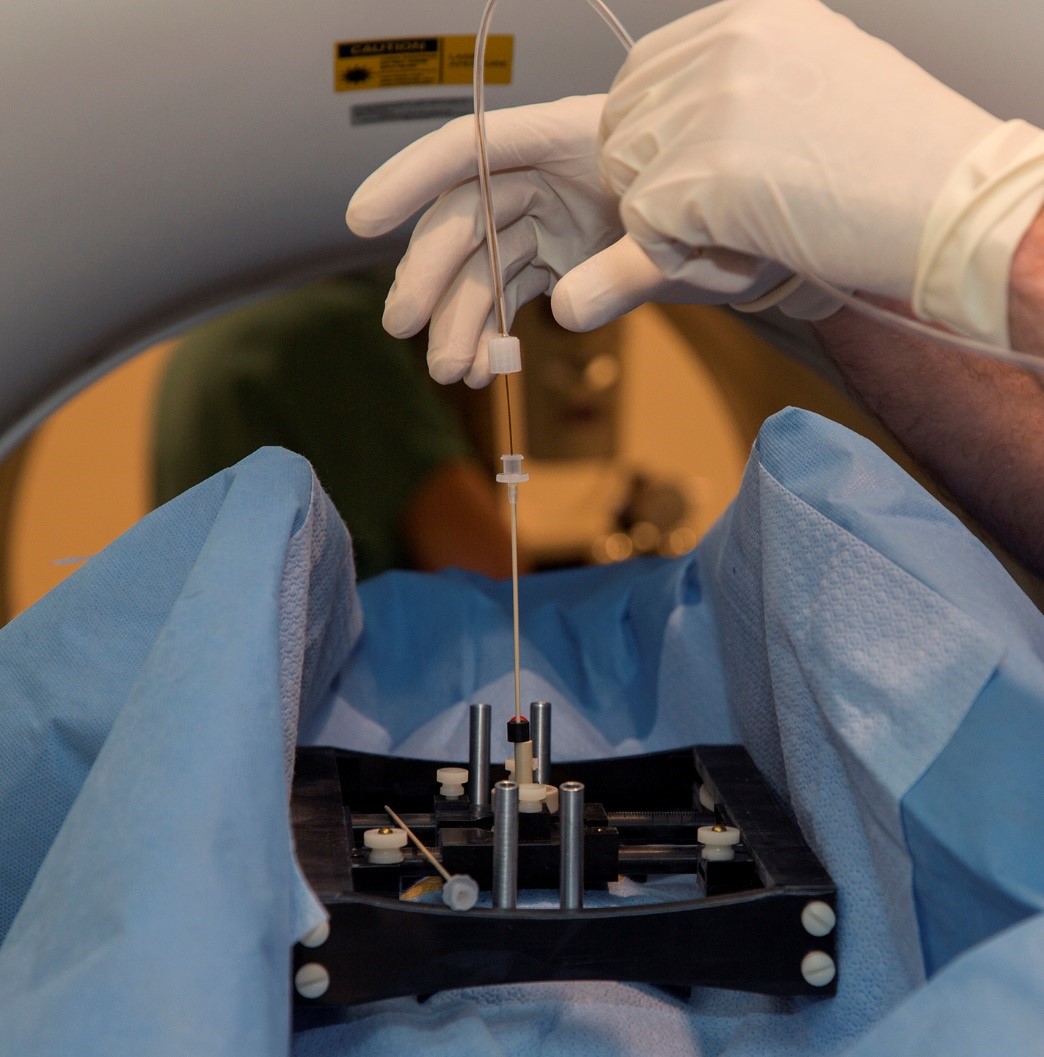
Dr. Boulis has been working on the latest improvements on his patient-mounted platform designed for spinal cord intraparenchymal injections. The goal of this project is to develop a platform that features fewer components and can be deployed as a single unit. It also considerably streamlines intra-operative assembly, reducing the surgical timeframe. Equally important, fewer individual parts in the system makes the attrition of components less likely in the multiple trips for cleaning and sterilization. Indications for its use include neurodegenerative disorders such as amyotrophic lateral sclerosis (ALS), spinal muscular atrophy (SMA), as well as chronic pain, spinal cord injury (SCI), multiple sclerosis (MS), spinal cord tumors, and transverse myelitis (TM).
Nerve Gap Repair
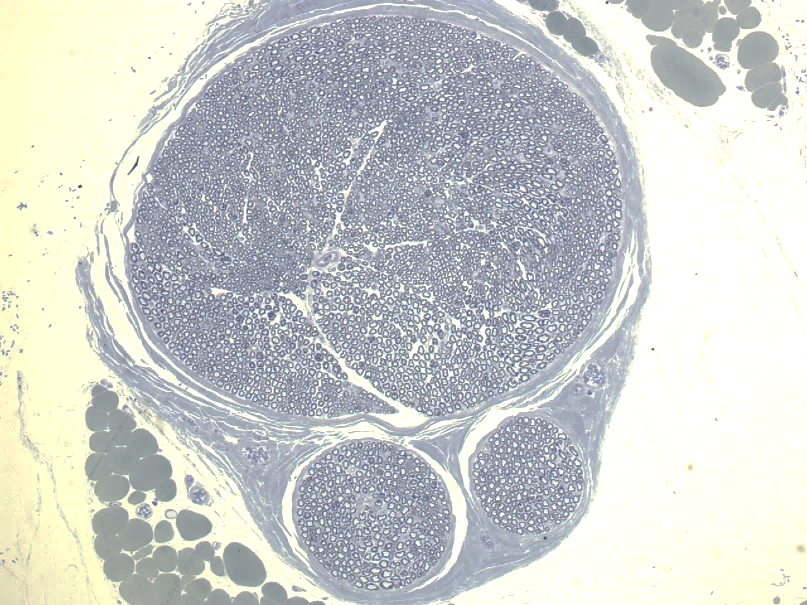
Traumatic injuries often include damage to the peripheral nerves. This causes loss of motor and sensory function in the affected body parts. When the damage is severe enough, the damaged region needs to be bypassed. Currently, the damaged portion of the nerve is repaired using nerves harvested from other portions of the patient’s body, known as autografts. However, this approach can cause loss of feeling and/or pain in the portions of the body innervated by those nerves. Therefore, alternative products are badly needed, particular for nerve defects larger than 3 cm. In partnership with Younan Xia at the Georgia Institute of Technology, we have been investigating the use of artificial conduits to repair injured nerves. Plain conduits support some nerve regeneration; however, they are quite inferior to autografts. These conduits can be augmented with a variety of factors, including artificially-induced Schwann cells (the cells that myelinate axons in peripheral nerves), neurotrophic factors (such as NT-3), and enzymes that reduce scar formation (such as ChABC). In one study, the combination of NT-3 and ChABC were tested under two release methods. Interestingly, a slow, continuous release of these proteins significantly improved the function of the repaired nerve, while pulsed release inhibited recovery. These results highlight spatial and temporal facets of these therapies can greatly impact their efficacy. In a second study, we compared conduits containing a single channel with conduits containing multiple channels. Having additional channels significantly improved the performance of the conduit. These advances are helping move conduits towards use in clinical practice.
Large Animal Models of Glioma
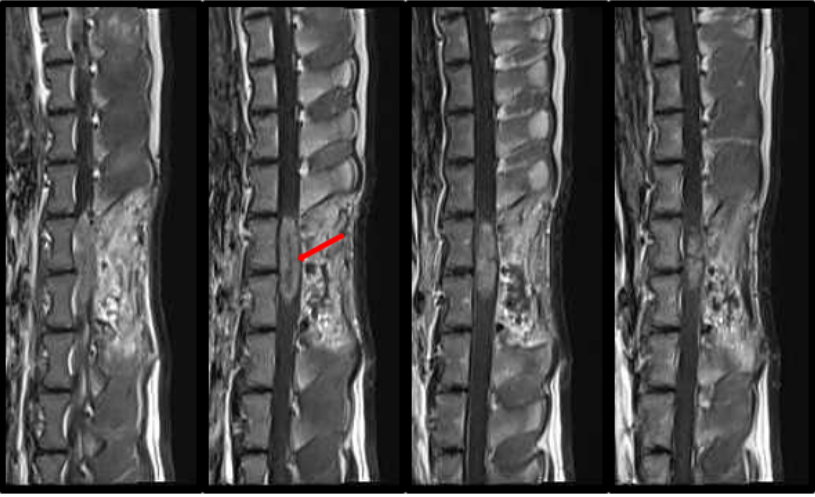
Glioma is a form of cancer of the brain and spinal cord. After years of research on gliomas and hundreds of clinical trials, the outcomes for patients with this form of cancer have not substantially improved. We believe that this is due to the limitations of the models of glioma that have been previously used to test novel therapies. Most models involved cells taken from patients with glioma. When injected into an immunosuppressed animal, these cells will grow and form a tumor. However, these tumors do not completely recapitulate the tumors observed in humans. Thus, drugs designed to kill these specific tumors may not be generalizable to all gliomas. In order to generate a more authentic model of this disease, we have developed gene therapy vectors that deliver oncogenes. When injected into the white matter tracts of the spinal cords of rats and pigs, these vectors produce gliomas in a matter of weeks that are indistinguishable from gliomas in humans. We believe that this model will serve as a superior testbed for new therapies for glioma. Current research in the lab is focused on modulating the disease severity and creating a brain glioma model.